Description
It differs from other powder testers in many ways but when assessing industrial value, three features are critical:
- The ability to simulate powder processing conditions, by testing samples in consolidated, moderately stressed, aerated or fluidised state
- The application of multi-faceted powder characterisation to assess dynamic powder flow, bulk and shear properties to construct the most comprehensive understanding of how a powder behaves
- Unparalleled sensitivity, enabling the differentiation of powders that other testers classify as identical
Features
- Fully automated test programs and data analysis
- Conditioning mode provides unparalleled repeatability
- Range of sample size, 10ml to 160ml (in addition a 1ml Shear Cell is available)
How It Works
The FT4 employs unique technology for measuring the resistance of the powder to flow, whilst the powder is in motion. A precision ‘blade’ is rotated and moved downwards through the powder to establish a precise flow pattern. This causes many thousands of particles to interact, or flow relative to one another, and the resistance experienced by the blade represents the difficulty of this relative particle movement, or the bulk flow properties.
Excellent reproducibility and sensitivity, this is achieved by moving the blade in a precise and reliable way. The advanced control systems of the FT4 accurately set the rotational and vertical speeds of the blade, which defines the Helix Angle and Tip Speed.
FT4 Powder Rheometer Methodologies
The FT4 is a truly universal powder flow tester, with four categories of methodologies, defined as Bulk, Dynamic Flow, Shear (in accordance with ASTM D7981) and Process.
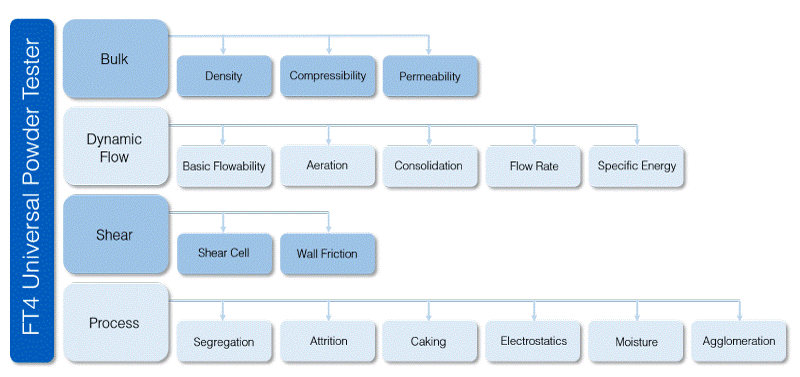
Proven Applications
The FT4 has application in all powder processing industries, including Pharmaceuticals, Fine Chemicals, Food, Cosmetics, Toners, Metals, Ceramics, Plastics, Powder Coatings, Cements and Additive Manufacturing. Applications extend to:
- Die / Capsule Filling
- Tablet Compression
- Hopper Flow
- Wet Granulation End Point & Scale Up
- Flow Additive Selection & Optimisation
- Humidity Effects
- Electrostatic Change
- Mixing / Blending
- Feeding
- Segregation
- Attrition
- Dry Powder Inhalers
- Caking
- Milling
- Conveying
- Wall Friction & Adhesion
- Hopper Design
- Compact Hardness & Payoff
- Vacuum Filling
- Agglomeration
Whether your objective is to optimise a formulation in a development environment, predict in-process performance, understand batch differences, or to ensure the quality of raw materials or intermediates, the FT4 will provide valuable and unique information that will help you address your powder flow challenges.
Bulk properties are not a direct measurement of flowability or shear, but nevertheless influence process performance and product attributes. The FT4 Powder Rheometer® measures three types of bulk powder properties: –
Density
Density defines the relationship between mass and volume. In principle this seems a simple concept, but the nature of powders means that their packing structure can change easily and significantly. Therefore when defining density, it is essential to ensure the packing state is well known and can be reproduced. This is achieved on the FT4 using a Conditioning cycle. When combined with other features such as the built in balance and Split Vessels, which allow a precise volume to be attained, the Conditioned Bulk Density can be measured with unprecedented levels of accuracy.
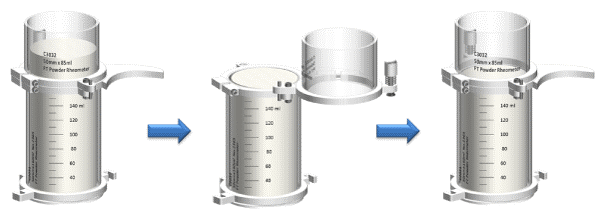

Compressibility
The measurement of Compressibility is achieved by applying increasing levels of compressive force with a piston to a conditioned powder and measuring the change in volume as a function of the applied load. The Vented Piston ensures that air trapped within the powder is able to readily escape, and the high resolution of the position measurement system allows for precise definition of Compressibility, expressed as a percentage change in volume for a given applied normal stress.
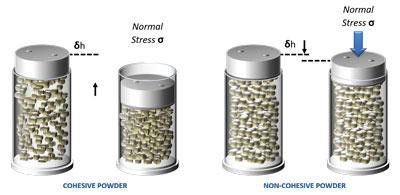
Compressibility = percentage change in volumn after compression (%)
Alternatively this data can be represented as a Compressibility Index or as Bulk Density, both as a function of applied normal stress.
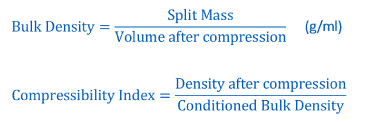
Permeability
Permeability is a measure of the powder’s resistance to air flow. Not to be confused with an Aeration test, this method utilises the vented piston to constrain the powder column under a range of applied normal stresses, whilst air is passed through the powder column. The relative difference in air pressure between the bottom and the top of the powder column is a function of the powder’s permeability. Tests can be completed under a range of normal stresses and air flow rates.
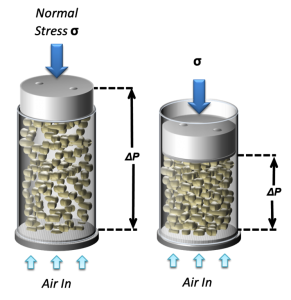
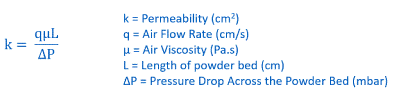
This important material property is relevant in many applications, including tabletting and filling, for example. In a tabletting process, the efficiency of air removal during the compression step will influence the mechanical properties of the compact, and should air be retained within the tablet due to low powder permeability, capping or lamination may occur. Within a filling application, the ability of the air to “back flow” out of the die or container through the powder as it enters depends on the bulk permeability and this will influence fill rate and fill consistency. Whilst permeability is a relatively simple bulk property, it is important in many processes and applications and should be accurately measured.
The Importance of Conditioning
Anyone who has worked with powders will know how easily they change their density, just as a result of handling them. Tip them from a beaker and they aerate, or tap the beaker on the bench and observe a reduction in volume as the powder becomes compacted.
These changes in density are a consequence of changes in the stress applied to the powder. As discussed previously, variation in stress level is likely to have a major impact on how the powder behaves, within a process or application, but also during a measurement. It is therefore essential to ensure the powder is prepared for any test by first establishing a uniform stress in the powder bed and eliminating pockets of air or localised compaction.
This preparation step is called Conditioning and is a simple, but effective mechanical process designed to prepare the sample for the following measurement. Utilising the same unique technology that is used in the dynamic methodologies (see previous pages), the Conditioning process involves gentle displacement of the whole sample in order to loosen and slightly aerate the powder. The aim is to disturb and gently drop each particle in order to construct a homogenously packed powder bed, removing any precompaction or excess air and ensuring the results from the following test are independent of how the operator handles the powder and places it into the testing vessel.
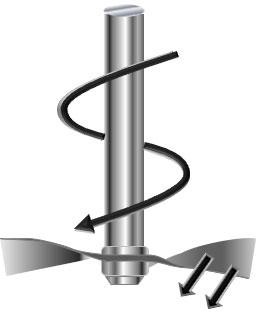
Powder Conditioning with Blade
A conditioning cycle is usually completed prior to every test in order to remove the variability introduced by the operator during loading of the sample, and any residual compaction from previous tests. The exception is where an intentionally consolidated sample is being evaluated, in which case conditioning is not employed.
The FT4 Powder Rheometer employs unique technology for measuring the resistance of the powder to flow, whilst the powder is in motion. A precision ‘blade’, or impeller, is rotated and moved downwards and upwards through the powder to establish a precise flow pattern. This causes many thousands of particles to interact, or flow relative to one another, and the resistance experienced by the blade represents the difficulty of this relative particle movement, or the bulk flow properties. The more the particles resist motion and the harder it is to get the powder to flow, the more difficult it is to move the blade.
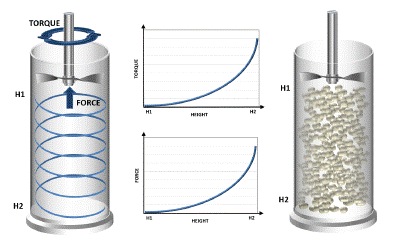
As the blade moves through the sample, the FT4 measures both rotational and vertical resistances, in the form of Torque and Force respectively. It is important to capture both signals as it is the composite of these two values that quantifies the powder’s total resistance to flow.
Using the calculation of WORK DONE, it is possible to represent both the Torque and Force signals as a Total Flow Energy, the energy required to move the blade through the sample from the top to the bottom of the powder column. However, because the values of torque and force are constantly changing, it is necessary to calculate the energy for each small distance travelled. This is the calculation of Energy Gradient, the energy measured for each millimetre of blade travel, expressed in mJ/mm.
Work Done = Energy = (Resistance x Distance travelled)
where ‘RESISTANCE’ is the combined Torque and Force
Energy Gradient = Energy per mm of blade travel
Calculating the area under the Energy Gradient curve provides the Total Flow Energy, representing the powder’s resistance to being made to flow in a dynamic state.
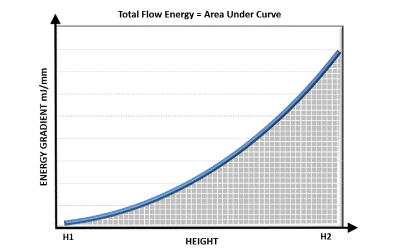
Energy Gradient is calculated directly from the measurements of Torque and Force
Confined and Unconfined Powder Flow
Two types of powder flow pattern are typically employed for quantifying flowability: –
Forced (or confined) Flow
A measure of the powder’s flowability when forced to flow, such as through a screw feeder or in an active feed frame. This property is defined as the Basic Flowability Energy, BFE, and is measured during the downward blade movement. The powder is confined by the closed bottom end of the test vessel.
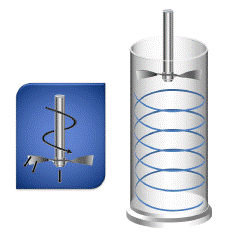
Direction of movement downwards
Low Stress (or unconfined) Flow
A measure of the powder’s flowability when unconfined, such as during low stress filling, or low shear blending. This property is defined as the Specific Energy, SE. In this measurement, the resistance to flow is measured as the blade traverses from the bottom of the vessel to the top. As there is no solid surface at the top of the vessel preventing the powder from dilating and moving upwards, the powder is unconfined during this test.
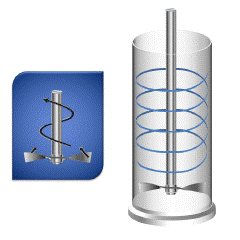
Direction of movement upwards
The regimes of confined and unconfined flow are very different and so it is important, when correlating data with process performance, to identify which regime is most representative of the process being considered.
Powder flow properties are complex and cannot be quantified by a single number. Flowability must be considered in relation to the conditions imposed by the process and application. Powders may exhibit “good” flow if loosely packed, but “bad” flow after consolidation. Some powders may flow well as long as flow rates are relatively high, however they may stop flowing when moved more slowly.
The FT4 Powder Rheometer has been designed to allow the effect of each of these External Variables to be investigated. By closely simulating process conditions in the measurement cell, the powder’s response to each variable can be quantified.
External variables include:
- Conditioning
- Aeration
- Flow (Shear) Rate
- Moisture
- Electrostatic Charge
- Storage Time
Using Dynamic Methods to Quantify the Effects of External Variables
Basic Flowability Energy is a measure of a powder’s flow properties when the powder is in a loosely packed state (following conditioning). Using this same dynamic methodology, it is possible to quantify how powder flow properties change when it is subjected to any of the external variables (defined in the previous sections).
Aeration
In order to quantify the influence of air, a controlled air supply can be introduced through a porous mesh at the base of the powder column. This method is not just to simulate processes and applications where air is intentionally introduced into the powder, such as during conveying, drying and in dry powder inhaler applications, but importantly to explore the cohesive forces that exist between particles.
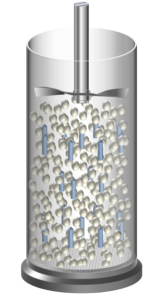
Air In Aeration Test
Cohesive forces are notoriously difficult to measure, but can now be accurately and directly quantified by assessing how aeration changes the flow properties of the bulk powder. Cohesive forces are a combination of Van der Waal’s and electrostatics, and tend to “bond” particles together. The introduction of air to the powder column attempts to separate adjacent particles and overcome these cohesive forces. If the forces are weak, each particle will become mechanically separated from its neighbour and the powder will become fluidised. The measured resistance to flow, the Aerated Energy, AE quantifies the strength of the cohesive forces.
For powders with weak cohesive forces, the Aerated Energy tends towards zero as the powder becomes fully aerated. Powders with moderate to high cohesion will exhibit a reduction in flow energy when aerated, but to a much lesser extent. In these cohesive powders, the tensile forces are too strong for the air to overcome and the particles do not separate. Instead a channel is established in the powder though which the air passes, and the corresponding Aerated Energy remains relatively high, even at high air velocities.
Contrasting Basic Flowability Energy with Aerated Energy results in the Aeration Ratio, AR, where:
Aeration Ratioxx = Basic Flowability Energy / Aerated Energyxx = BFE / AExx
where ‘xx’ defines the air velocity in mm/s at which the Aerated Energy measurement is taken.
The Aeration Ratio is a measure of the powder’s sensitivity to aeration.
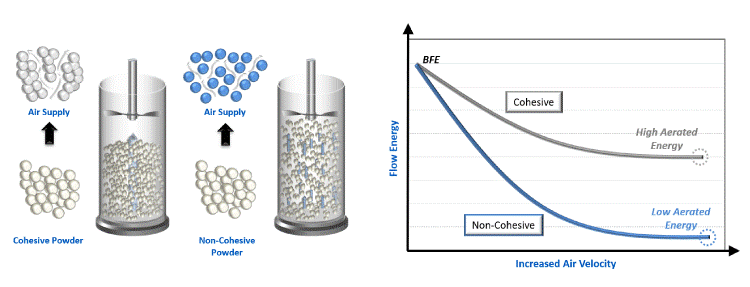
Consolidation
The effect of consolidation on flow properties can be directly quantified by the Consolidation Energy, CE. This is a very similar test to the Basic Flowability Energy, but is completed on a powder sample that has first been subjected to a level of consolidation. Like the BFE method, the flow energy is determined as the blade moves through the powder sample from the top to the bottom. Initially a Conditioning cycle is employed to generate a uniform packing density, before the powder is subjected to a number of taps, in order to induce consolidation in the sample, prior to it being measured. The resulting increase in resistance to flow is quantified by the Consolidation Energy.
It is also possible to consolidate the powder using an applied normal force. This compaction technique more closely simulates how powders would behave during storage, in contrast to behaviour during transport or in other environments where they are subjected to vibration. In both methods, the powder is first consolidated before the flow energy is measured.
Contrasting the Consolidated Energy with the Basic Flowability Energy provides a relative change in powder flow properties as a function of consolidation. It is a measure of the powder’s sensitivity to consolidation and is expressed as the Consolidation Index, CI, where:
Consolidation Index, CIxx = Consolidation Energyxx / Basic Flowability Energy = CExx / BFE
where ‘xx’ defines either: –
- The number of taps, or
- The applied normal stress during compaction (kPa)
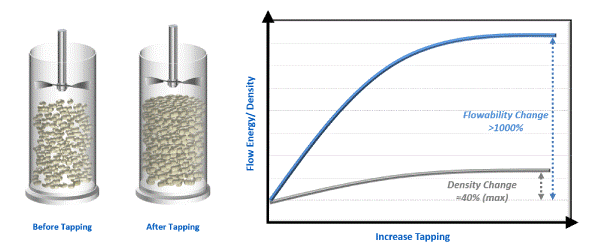
Traditional tapped density based methods, such as Carr’s Index and Hausner Ratio, seek to define certain aspects of powder flowability based on a volume change, as a result of tapping the sample. As the graph above illustrates, changes in density may be as high as 40%, however flowability may actually have changed by 1000% as a consequence of tapping. This direct measurement of Consolidated Energy quantifies that the flow properties are in fact 10 times worse after tapping and illustrates why inferring flow characteristics from density measurements is often misleading. After all, from a processing and application perspective, the interest is mostly to determine whether the product will flow, rather than whether its density has changed.
Flow (Shear) Rate Sensitivity
In addition, powders with high flow rate sensitivity will require an optimised and specific mixing configuration if blend uniformity is to be attained. The advantage of powders with low flow rate sensitivity is that low shear mixing operations can be employed, whilst still ensuring homogeneity, minimising the chances of particle attrition and electrostatic charge evolution which are commonplace in high shear blending. Powders exhibiting high flow rate sensitivity normally require high shear processes in order to blend efficiently.
Powders typically exhibit different flow behaviour when moved at different flow rates. This means that they may flow freely at one speed and badly at another. This sensitivity to changes in flow rate has a number of implications for powder processors and can seriously effect process stability.
Unlike many liquids, powders are rarely Newtonian in nature, exhibiting a complex behavioural relationship with the speed at which they flow. In fact it is common that powders are more difficult to move at lower speeds than at higher velocities, meaning that if a process is subject to variation in the rate powder moves through it, blockages may occur should flow rates drop below a critical value.
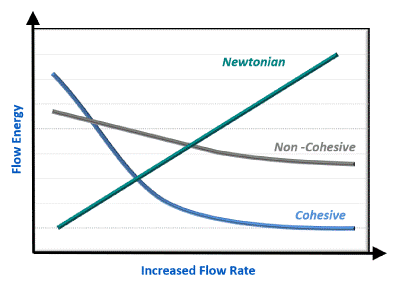
Utilising the dynamic methodologies of the FT4 Powder Rheometer, and good experimental design, it is possible to investigate a number of other behavioural properties of powders. These include: –
Caking
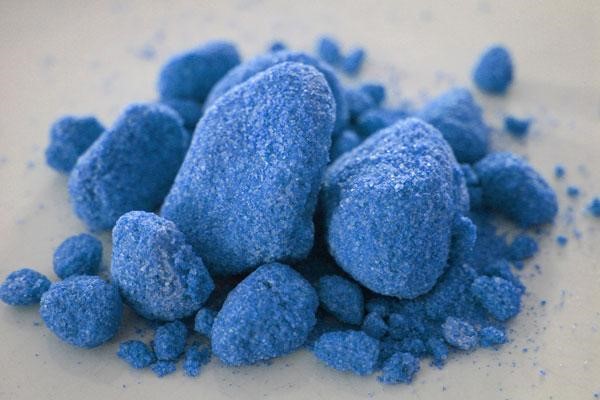
Powder consolidation with time
Moisture
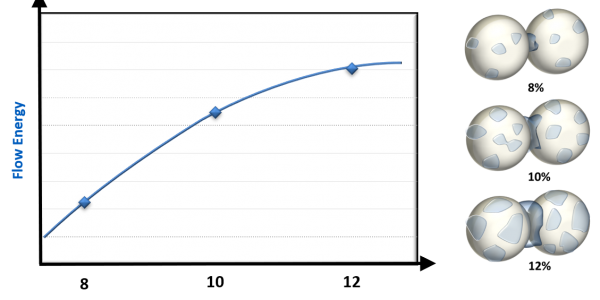
The influence of free moisture on the behaviour of the powder
Segregation
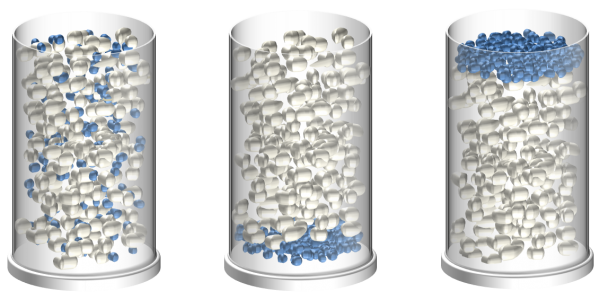
The potential for particles to rearrange according to their size / density
Attrition
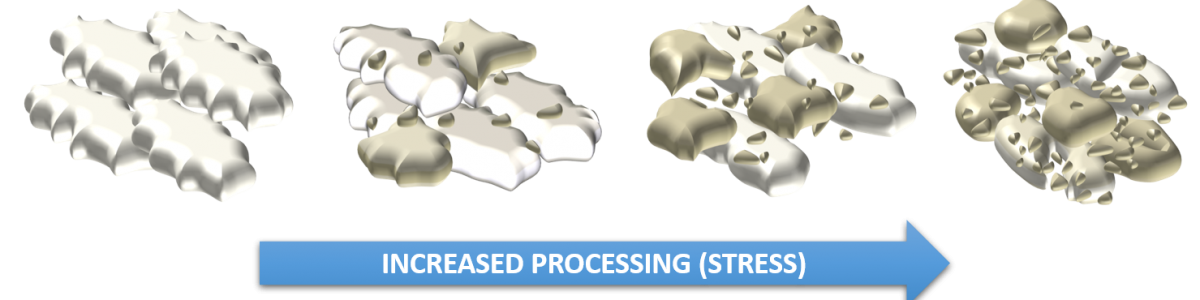
Particle friability, resulting in changes in particle size, shape and surface area
Electrostatics
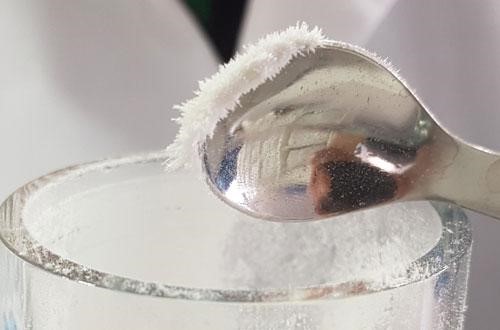
Changes in behaviour as a function of electrostatic charging
Agglomeration
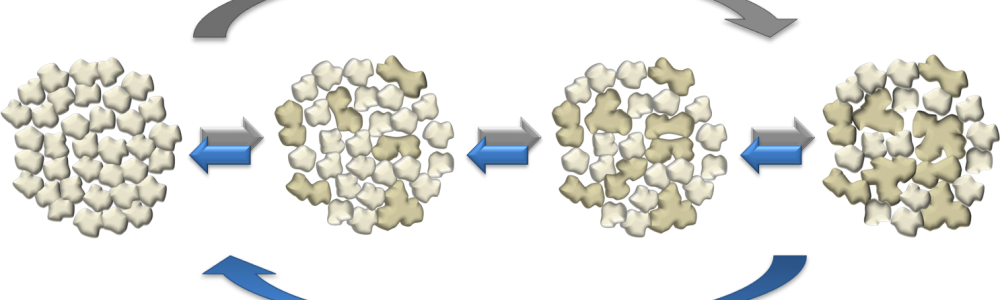
The formation of agglomerates from primary particles, usually as a function of being processed
The FT4 Powder Rheometer® also includes a Shear Cell accessory which allows the powder’s shear properties to be quantified. The technique has been published by the ASTM International Committee D18 on Soil and Rock. Shear testing is a very different technique to that of dynamic testing, and always characterises the powder in a consolidated state. It is also a fairly static test, measuring powder behaviour as it transitions from no-flow to flow.
For these reasons, it is clear to see why shear cells are ideally suited to predicting powder behaviour in process operations where the powder is consolidated and where flow rates are low and / or sporadic. They have been successfully applied for understanding powder behaviour in hoppers, and also provide some of the data needed to carry out a hopper design exercise (based on Jenike stress theory developed during the last century). However, as a consequence of the regime in which they operate, they are less suited to predicting powder behaviour in low stress or dynamic applications, such as mixing, filling, feeding and conveying.
Operating Principle
At very low speeds, a shear (or horizontal) force is applied to an upper layer of powder whilst the adjacent lower layer is prevented from moving (or vice versa). The force continues to increase but no relative movement at the shear plane occurs until the shear force is sufficiently high to overcome the powder’s shear strength, at which point the powder bed ‘yields’ and the upper layer of powder slips against the lower.
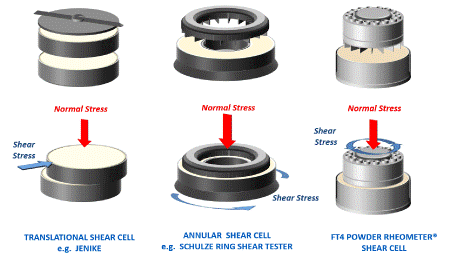
Several types of shear cell design have emerged over the last 50 years, however each employs the same concept of shearing one layer of powder against the other. The designs most frequently in use today are rotational in nature, and are preferred as they allow the two layers of power to be sheared relative to one another over a large distance, in contrast to translational designs that quickly exhaust their range of displacement.
Each design has its strengths and weaknesses, and all types remain in use today. For a detailed comparison of features, benefits and weaknesses, please contact us directly for further information.
In a typical shear cell test sequence, several shear tests would be carried out at different levels of normal stress. The data produced represents the relationship between shear stress and normal stress, which can be plotted to define the powder’s Yield Locus. In simple terms, the higher the shear stress for a given normal stress, the less likely it is that the powder will yield and begin to flow when confined under a similar consolidation stress in a hopper or other vessel.
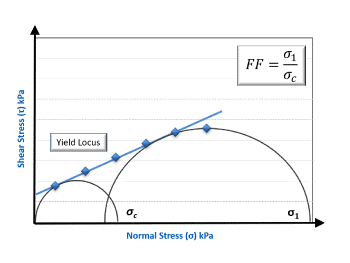
It is possible to apply a number of mathematical models to this data, but it is important to consider that in doing so, trends may be exaggerated or reduced. Fitting Mohr stress circles to the yield locus identifies the Major Principle Stress (Sigma 1) and Unconfined Yield Strength (Sigma c), and the ratio of the former to the latter quantifies the Flow Function, FF. Flow Function is a parameter commonly used to rank flowability, with values below 4 denoting poor flow and above 10, good flow.
Wall Friction
The Wall Friction test provides a measurement of the sliding resistance between the powder and the surface of the process equipment. This is particularly important for understanding discharge behaviour from hoppers, continuity of flow in transfer chutes and tablet ejection forces. It is also useful when investigating whether a powder will adhere to the wall of process equipment and various other surfaces, such as the inside of sachets, capsules and other packaging material.
The measurement principle is very similar to the shear cell test, but rather than shearing powder against powder, in this test a coupon of material representing the process equipment wall is sheared against the powder in question. The FT4 Wall Friction accessory allows for a range of coupons to be investigated, and bespoke surfaces can be manufactured if required.
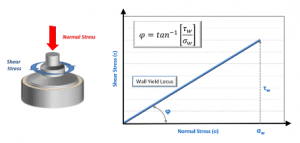
Data is typically represented as a plot of shear stress against normal stress, allowing the determination of Wall Friction Angle (phi). The greater the wall friction angle, the higher the resistance between the powder and wall coupon.
This data can be utilised in specific studies, as outlined above, but is also required as part of a hopper design exercise.
Hopper Design
Hoppers are used extensively throughout the processing environment and whilst they are often considered to be simple systems, they are responsible for causing a great deal of process interruption and product quality issues.
If a powder possesses properties that are not optimised for the hopper geometry and equipment surface, then flow from the hopper may be variable or even none existent. However, since the pioneering work carried out by Andrew Jenike in the mid twentieth century, it has been possible to utilise data from shear cell and wall friction tests to calculate the critical hopper dimensions to ensure good flow.
The FT4 comes with fully automated hopper design software, which takes the results directly from shear cell and wall friction tests and runs the data through the hopper design algorithms. The result is a fully automated hopper design exercise in less than 3 hours.
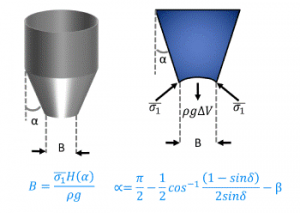
Today, this hopper design process remains one of the very few fundamental approaches to equipment design, based on an understanding of material properties and the stress regime within the equipment. Unfortunately, such an approach is not available for mixers, feeders, conveyers, dryers, compression processes or any of the other unit operations routinely used in powder processing.